In my previous post, Where is the 1919 Data Proving General Relativity?, I explained that the photographic plates of the 1919 eclipse-observation expeditions, to Príncipe, off the coast of Africa, and to Sobral, in northeastern Brazil, sponsored by the Royal Astronomical Society, have gone missing. This is not in itself a falsification of the theory of general relativity, but it is certainly damning circumstantial evidence.
Now, the 1919 expeditions were certainly not the only such eclipse-observing expeditions, but the results of other similar expeditions have been disappointing, to say the least. One of the limiting factors of such expeditions is that the longest possible total solar eclipse on Terra (the Earth) is about seven and half minutes, and most are shorter, and it is rare that observation conditions in hard-to-reach locations are optimal. Here is a quick summary from Clifford Will, author of Was Einstein Right? Putting General Relativity to the Test1, of the results of subsequent expeditions undertaken in the following half-century.
Although the eclipse results distinguished clearly among the possibilities of no deflection, the Newtonian deflection, and the Einsteinian deflection, their relatively large experimental errors made it important to repeat the measurements. During subsequent eclipses, numerous expeditions attempted to do better—there were three expeditions in 1922, one in 1929, two in 1936, one each in 1947 and 1952, and most recently, one in 1973. Surprisingly, there was very little improvement, with different measurements giving values anywhere between three-quarters and one and one-half times the general relativistic prediction. The 1973 expedition is a case in point. Organized by the University of Texas and Princeton University, the observation took place in June at Chinguetti Oasis in Mauritania (eclipses have the nasty habit of occurring in out-of-the-way places). The observers had the benefit of 1970s technology: Kodak photographic emulsions, temperature control inside the telescope shed (the outside temperature at mid-eclipse was 97°F), sophisticated motor drives to control the direction of the telescope accurately, and computerized analysis of the plates. Unfortunately, they couldn't control the weather any better than Eddington could. Eclipse morning brought high winds, drifting sand, and dust too thick to see the Sun. But as totality of the eclipse approached, the winds died down, the dust began to settle, and the astronomers took a sequence of photographs during what they have described as the shortest six minutes of their lives. They had hoped to gather over 1,000 star images, but the dust cut the visibility to less than 20 percent and only a disappointing 150 were obtained. After a follow-up expedition to the site in November to take comparison plates, the photographs were analyzed using a special automated device called the GALAXY Measuring Engine at the Royal Greenwich Observatory near London. The result was a deflection of 0.95 ± 0.11 times the Einsteinian prediction, still only a modest improvement over previous eclipse measurements. [Will, pp.79-80, my emphasis]
With a number of expeditions providing different measurements giving values anywhere between three-quarters and one and one-half times the general relativistic prediction, it is hard to argue that the Einstein prediction of how much light will bend as it passes near Sol (the Sun) has been proven.
Fortunately, a new technique has been developed, namely the measurement of quasar occultation [hiding] by Sol.
The 1973 expedition was really the swan song for this type of measurement, because much more accurate determinations of the deflection were already being made using a technique that was a marriage of two of the most important astronomical discoveries of the twentieth century: the radio telescope and the quasar. [Will, p.80]
First, let us read what Will wrote about the discovery of quasars, their high redshifts, and the determination that they are the most distant objects in the visible universe.
The discovery of quasars thrust general relativity immediately to the forefront of astronomy. The reason was an energy crisis of truly cosmic proportions. Within a few years after the discovery of the quasar associated with 3C48, it was found that it and other quasars like it were among the most distant objects in the universe. What the astronomers thought were unusual spectra were actually rather ordinary spectra in which all features were shifted uniformly to the red end of the spectrum. This meant that the quasars must be moving away from us at high speed, 30 percent of the speed of light in the case of 3C48. The shift in wavelength to the red is a consequence of the Doppler shift, the same effect that, in the case of sound, causes a railroad crossing bell to have a lower pitch when we are moving away from it. In 1929, the astronomer Edwin Hubble had discovered that the systematic “red shift” of the spectra of distant galaxies, first observed fifteen years earlier by Vesto M. Slipher, had a pattern associated with it, so that the farther the object, the larger its recession velocity (in almost direct proportion), and the larger the red shift of its spectral features. Since that time, it was accepted that the universe is expanding uniformly in all directions. For 3C48, for instance, the recession velocity of one-third of the speed of light corresponded to a distance of about 6 billion light years. The light that we see today from 3C48 left it when the universe was two-thirds as old as it is now. Because the quasars were so distant, one would have expected them to be faint, yet they were very bright sources, both in visible light and in radio waves. Therefore their intrinsic brightness or luminosity must be enormous. For 3C48, the numbers translated into 100 times the brightness of our own galaxy. [Will, pp.7-8, my emphasis]
So the idea is that the light emitted by quasars is highly redshifted, and since it is supposed that highly redshifted celestial objects are the most distant, and moving away from us with the highest velocities, their light can be considered to be coming towards us as a point source.
Now, to undertake a set of observations using quasars to estimate how much light, here radio waves, bends as it passes close to Sol, at least two quasar sources are needed, as Will explains below.
We also need a very sharp source of radio waves. Most astronomical sources are unsuitable for this purpose because they are extended in space. For example, most radio galaxies emit their waves from an extended region that can be as large as a degree. The discovery of quasars, besides motivating applications of general relativity to astrophysics, provided the ideal source of radio waves to test the deflection of light. Because they are so distant, on the order of 10 billion light years away, they appear much smaller in extent, making it possible to pinpoint their location more accurately. Yet despite their distance, many of them are powerful radio sources. However, a powerful point source of radio waves is not the only ingredient for a successful light deflection experiment. We need at least two of them fairly close to each other on the sky, and they have to pass near the Sun as seen from Earth. We need at least two for the same reason as we needed a field of stars behind the eclipsed Sun in the optical deflection measurements: the stars whose images are far from the Sun are used to establish the scale because their light is relatively undeflected, and the movement of the star images close to the Sun is used to determine the deflection. [Will, p.82, my emphasis]
Now it turns out that there is a pair of quasars, named 3C273 and 3C279, that pass (visually) close to Sol every year, so it is possible, using large telescopes stationed in their normal conditions, to make observations that are not affected by inclement weather, and without being subject to an approximately six-minute window.
Now comes a heavenly coincidence. Two of the brightest quasars on the sky are 3C273 and 3C279 and both have a small angular size. Each October 8, they pass very close to the Sun as seen from Earth; in fact, 3C279 actually passes behind the Sun, while 3C273 comes as close as 4°…. The power of radio interferometry is that it is most adept at measuring the angle between two such radio sources, with even more accuracy than it can measure their absolute positions on the sky. Over a period of ten days centered on October 8, the pair of quasars passes from one side of the Sun to the other, and each day the angle between the two can be measured on the radio interferometer. What would be the expected output of the instrument? Initially, when both quasars are far from the Sun, the angle measured would be the true, undeflected angle between them. As days pass, the pair approaches the Sun, and by October 7, 3C279 is much closer than 3C273. Because the amount of deflection varies inversely as the distance of the light path from the Sun, the radio waves from 3C279 are deflected more than those from 3C273, and the angle between the two will appear to increase. As 3C279 just grazes the Sun, its light is deflected by about 1.75 arcseconds, while 3C273, 9° away and therefore 35 times farther from the Sun, is deflected only 0.05 arcseconds. By October 9, 3C279 is on the same side of the Sun as 3C273, so the angle between the two appears to decrease. As days continue to pass, the pair moves farther from the Sun and their separation returns to the original value. [Will, pp.100-101, my emphasis]
There is no doubt that the results from quasar occultation observations are far more accurate and predictable than could possibly made with solar eclipse observations.
Nevertheless, the quasar occultation observations are only reliable if we accept that the light is coming from great distances, which is assumed because of the high redshifts of 3C273 and 3C279.
It is time to examine Eric Lerner’s The Big Bang Never Happened2 to better understand the origins of the expanding-universe hypothesis and of the assumption that highly-redshifted celestial objects are distant.
Carl Wirtz, a German astronomer, put all the forty-odd observations together in 1924 and noted a correlation—the fainter the galaxy the higher its redshift, thus the faster it is receding. Assuming that fainter galaxies are more distant, then velocity increases with distance. The conclusion was tentative, since the distances to the galaxies were uncertain. But the American astronomer Edwin Hubble and his assistant Milton Humason soon began to examine Wirtz’s findings. Hubble had developed a new way of measuring the distance to a galaxy, based on the known brightness of certain peculiar stars called Cepheid variables. Soon word filtered through the astronomical community that Hubble’s data seemed to confirm the relation between redshift and distance.
This news was of immense interest to a young Belgian priest and budding relativist, Georges-Henri Lemaître. Born in 1894, Lemaître received his doctorate in physics in 1920, and shortly thereafter entered a seminary to study for the priesthood. While at the Seminary of Maline, he became fascinated with the new field of general relativity, and after being ordained in 1923, went to England to study under Eddington. He then spent the winter of 1924-1925 at Harvard Observatory, where he heard Hubble lecture, and learned of the growing evidence for the redshift- distance relation.
In the next two years Lemaître developed a new cosmological theory. Studying Einstein’s equations, he found, as others had before him, that the solution Einstein proposed was unstable; a slight expansion would cause the repulsive force to increase and gravity to weaken, leading to unlimited expansion, or a slight contraction would, vice versa, lead to collapse. Lemaître, independently reaching conclusions achieved five years earlier by the Russian mathematician Alexander Friedmann, showed that Einstein’s universe is only one special solution among infinite possible cosmologies—some expanding, some contracting, depending on the value of the cosmological constant and the “initial conditions” of the universe.
Lemaître synthesized this purely mathematical result with Wirtz’s and Hubble’s tentative observations, and concluded that the universe as a whole must be expanding, driving the galaxies apart. And if the universe is expanding, then any of the cosmological scenarios that led to expansion could be a valid description of the universe. But cosmic repulsion and gravity are not delicately balanced—repulsion predominates in an expanding universe.
Lemaître put forward his hypothesis of an expanding universe in a little-known publication in 1927, and within two years his work and Friedmann’s had become widely known and accepted in the tiny cosmology fraternity. By this time, 1929, Hubble had published the first results showing the redshift relation, apparently confirming Lemaître’s idea of an expanding universe. [Lerner, pp.133-134]
So the hypothesis that highly redshifted celestial objects are distant presupposes the idea that we live in an expanding universe, which Alexander Friedmann (1888-1925) and Georges Lemaître (1891-1966)—the latter a student of Arthur Eddington (1882-1944) of Príncipe fame—had proposed as a consequence of Einstein’s theory of general relativity.
Wait a minute! The logical progression works as follows:
Assume that the theory of general relativity is valid;
Derive the theory of an expanding universe from (1) based on the varying redshifts of galaxies;
Discover quasars, which are high-redshifted objects;
Assume they are distant, from (2);
Make quasar occultation observations;
Determine that the radio waves coming from the occulted quasar are bent according to the predictions that follow from general relativity;
Affirm that quasar-occultation observations confirm general relativity.
This is called circular reasoning: assume general relativity to be valid in order to confirm general relativity!
But what if 3C273 and 3C279 are not as distant as is supposed by the mainstream?
I checked in Halton Arp’s (1927-2013) Quasars, Redshifts and Controversies3 and his Seeing Red: Redshifts, Cosmology and Academic Science4 about 3C273 and 3C279. Reading these, there is no question that both quasars are in the neighboring Virgo cluster.
Already in 1966, Arp had determined that quasar 3C273 and radio galaxy 3C274 are at similar distances inside the Virgo Cluster, which is, according to Wikipedia5, estimated at some 53.8 ± 0.3 Mly, which is quite a bit closer than “the order of 10 billion light years away” that Will uses above.
Here is Arp explaining the relationship between 3C273 and 3C274:
What could have been ejected from the central galaxy of the Virgo Cluster? I had stumbled across the answer in 1966 while finishing The Atlas of Peculiar Galaxies…. aligned directly across M49 (#134 in the Atlas), roughly north-south, are two of the brightest radio sources in the sky, 3C273 and 3C274 (M87). The improbability of this being a chance association was computed in 1967 to be about one in a million. Perhaps even more convincing was the common-sense question: Is it significant that the brightest radio quasar in the sky falls in the dominant cluster in the sky—and forms a pair with the brightest radio galaxy in the cluster, almost exactly aligned across the brightest galaxy in the center of the cluster? This result was published in Science in 1966 and Astrophysical Journal in 1967. It is incomprehensible to me how the field could have gone on believing quasars were at their redshift distances after even this one single result. More than 30 years ago astronomy took a gamble, against odds of a million to one, that this observation was an accident. [Seeing Red, p.117, my emphasis]
Arp states that
astronomers insisted that [3C273’s] redshift placed it at 54 times greater distance in the background. [Seeing Red, p.116]
Furthermore, Arp looks at the relationship between quasars 3C273 and 3C279, the ones that were referred to in Will’s book. It turns out that 3C279 is called a blazar because it is violently variable. The two are closely connected, as can be seen in the following image:
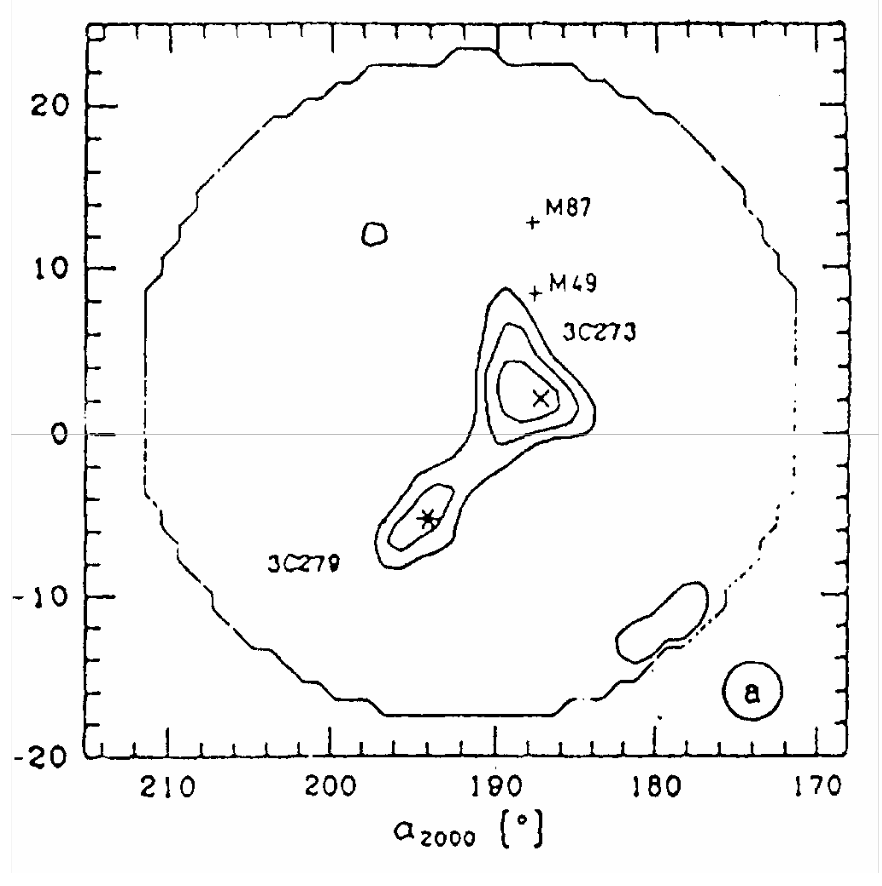
Arp continues:
Naturally, we could only deal with published data, so it was good fortune to find a published map of Virgo in the low energy gamma rays in the proceedings of a meeting held in March 1992. That map is shown here …. It is clear that 3C273 (z = .158) is linked to 3C279 (z = .538)…. it is clear that the very elongated isocontour lines between the two quasars in [the figure above] represent a real physical connection. [Seeing Red, p.132]
Arp concludes:
The quasar 3C279 is violently variable and was, from the Harvard patrol camera records in the early 1900’s, once one of the brightest apparent-magnitude quasars in the sky. Like 3C273 then, it is reasonable that it would be associated with the largest aggregate of bright galaxies and radio sources in the sky. It is classified as a “blazar,” and is one of the brightest gamma ray sources in the sky. [Seeing Red, pp.132-133]
So, according to Halton Arp, 3C273 and 3C279 are not billions of light years away, but tens of millions of light years away, in the neighboring Virgo cluster.
What this means is that the data from the quasar-occultation observations would need to be reëxamined with the changed assumption that the quasars are relatively close, and not remote. Given what happened to Halton Arp, who lost access to U.S.-controlled telescopes in the mid-1960s once he proposed that quasars were not necessarily distant objects, this reëxamination is unlikely to take place in the immediate future.
I must insist that neither what I wrote in this post nor what I wrote in the previous one falsifies general relativity. Nevertheless, it is clear that both posts raise interesting questions, and it is completely legitimate to be skeptical. I will continue to examine relativity, both special and general, in future posts.
If you wish to donate to support my work, please use the Buy Me a Coffee app.
Clifford M. Will. Was Einstein Right? Putting General Relativity to the Test. New York: Basic Books, 1986.
Eric Lerner. The Big Bang Never Happened: A Startling Refutation of the Dominant Theory of the Origin of the Universe. New York: Times Books, 1991.
Halton Arp. Quasars, Redshifts and Controversies. Berkeley, CA: Intersteller Media, 1987.
Halton Arp. Seing Red: Redshifts, Cosmology and Academic Science. Montreal: Apeiron, 1998.
Wikipedia. Virgo Cluster. https://en.wikipedia.org/wiki/Virgo_Cluster.
Begs a fascinating question across many sciences as to suppression of data refuting older opinion. There appears to be incentive by individuals in high places in the given science community to actively intervene to impede evidence. In Archeology we have seen this with dates found and denied for over 50 years regarding humans in North America. 250,000 YBP.: https://pleistocenecoalition.com/newsletter/january-february2016.pdf#page=2
Another great installment John!
What a vast tower of nonsense they have constructed on the shakiest of assumed foundations!
It reminds me of the way they try to teach Einstein's Gravity warping "space-time" with balls on a rubber sheet - ignoring the fact that this means the cause is created by the effect!
Halton Arp was a giant compared to these careerist imbeciles, but we've seen what happens to real thinkers when they start to question the True Faith of the Standard Model, they get sent to the naughty corner and have their telescopes and livelihoods taken away.
Most cosmologists even today would be unable to answer the question "and what is a Quasar?" and yet they assure you that they know exactly where they are and how they work and build a tower of pipedreams on it!!